Speakers of the International Symposium on Bioorganic Chemistry (ISBOC-11)
Keynote speakers
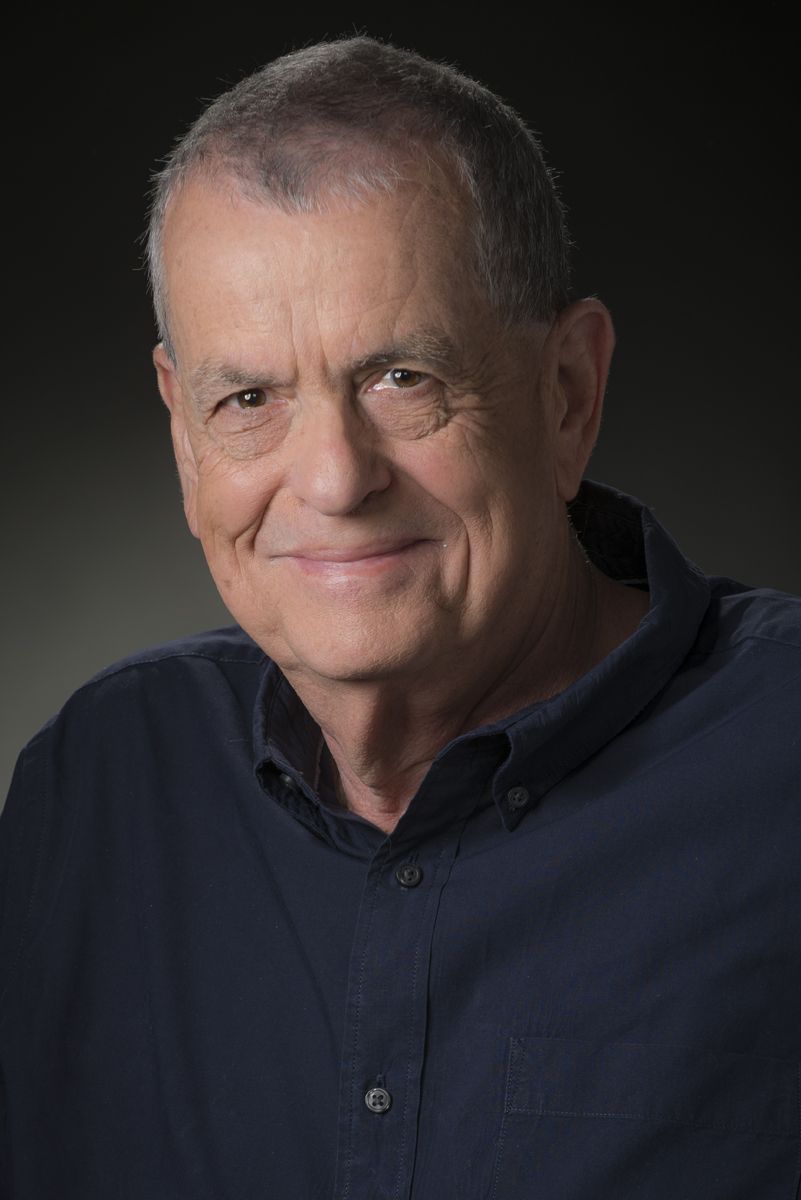
Aaron Ciechanover
Technion, Haifa, IL
- Talk
The Ubiquitin Proteolytic System - From Basic Mechanisms thru Human Diseases and on to Drug Development
Between the 50s and 80s, most studies in biomedicine focused on the central dogma - the translation of the information coded by DNA to RNA and proteins. Protein degradation was a neglected area, considered to be a non-specific, dead-end process. While it was known that proteins do turn over, the high specificity of the process - where distinct proteins are degraded only at certain time points, or when they are not needed any more, or following denaturation/misfolding when their normal and active counterparts are spared - was not appreciated. The discovery of the lysosome by Christian de Duve did not significantly change this view, as it was clear that this organelle is involved mostly in the degradation of extracellular proteins, and their proteases cannot be substrate-specific. The discovery of the complex cascade of the ubiquitin solved the enigma. It is clear now that degradation of cellular proteins is a highly complex, temporally controlled, and tightly regulated process that plays major roles in a variety of basic cellular processes such as cell cycle and differentiation, communication of the cell with the extracellular environment and maintenance of the cellular quality control. With the multitude of substrates targeted and the myriad processes involved, it is not surprising that aberrations in the pathway have been implicated in the pathogenesis of many diseases, certain malignancies and neurodegeneration among them, and that the system has become a major platform for drug targeting.
- About Aaron Ciechanover
Aaron Ciechanover was born in Haifa, Israel in 1947. He received his M.Sc. (1971) and M.D. (1973) from the Hebrew University in Jerusalem. After completing his national service (1973-1976) as a military physician, he continued his studies to obtain a doctorate in biological sciences in the Faculty of Medicine at the Technion (D.Sc.; 1982). There, as a graduate student with Dr. Avram Hershko and in collaboration with Dr. Irwin A. Rose from the Fox Chase Cancer Center in Philadelphia, USA, they discovered that covalent attachment of ubiquitin to a target protein signals it for degradation. They deciphered the mechanism of conjugation, described the general proteolytic functions of the system, and proposed a model according to which this modification serves as a recognition signal for a specific downstream protease. As a post- doctoral fellow with Dr. Harvey Lodish at the M.I.T., he continued his studies on the ubiquitin system and made additional important discoveries. Along the years it has become clear that ubiquitin-mediated proteolysis plays major roles in numerous cellular processes, and aberrations in the system underlie the pathogenetic mechanisms of many diseases, among them certain malignancies and neurodegenerative disorders. Consequently, the system has become an important platform for drug development. Among the numerous prizes Ciechanover received are the 2000 Albert Lasker Award, the 2003 Israel Prize and the 2004 Nobel Prize (Chemistry; shared with Drs. Hershko and Rose).
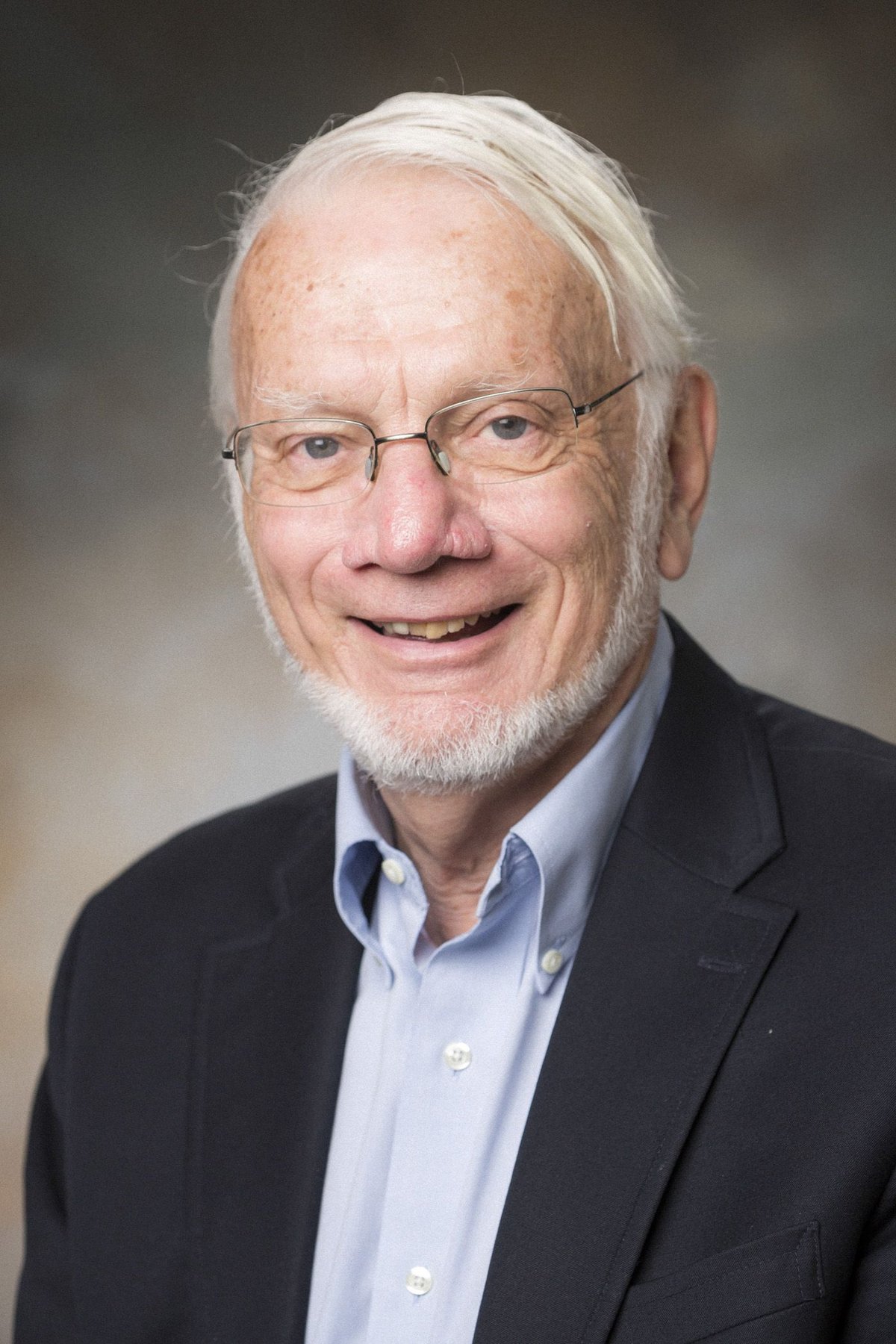
Thomas A. Steitz
Yale University, Connecticut, US
- Talk
The Structure and Function of the Ribosome Complexes with Various Protein Factors and Antibiotics
We have obtained many insights into the structural basis of ribosome function in protein synthesis from our structural studies of the large ribosomal subunit as well as the 70S bacterial ribosome, and their complexes with substrates, protein factors or antibiotics. These have elucidated the mechanism by which this ribozyme catalyzes peptide bond formation and the specificity and mode of its inhibition by antibiotics.
We have obtained the structure of the complex of the 70S ribosome with tRNAs and EF-G in a previously unseen compact conformation. This compact conformation of EF-G, unlike the elongated one, allows the simultaneous binding of a tRNA in the A site and EF-G. We propose that the conversion of the compact to the elongated conformations of EF-G is responsible for tRNA translocation. The structures of the 70S ribosome with the factor EF4 (LepA) with tRNA bound in the P site or in the A and P sites provide the first insights into EF4’s possible role in protein synthesis. Our structure of the 70S ribosome bound with a ribosome rescue protein (yaeJ) shows how it rescues stalled ribosomes.
The structures of some of our antibiotic complexes have been used by Rib-X Pharmaceuticals, Inc. (now Melinta Therapeutics) of New Haven to develop new potential antibiotic compounds that are effective against MRSA, one of which has successfully completed phase II clinical trials. Recently, we have obtained the structures of the 70S ribosome complexed with various oligopeptides that bind in the peptide tunnel, but in the opposite direction from peptides being synthesized; these structures might enable the creation of other new antibiotics.- About Thomas A. Steitz
Thomas A. Steitz is Sterling Professor of Molecular Biophysics and Biochemistry and Professor of Chemistry at Yale University as well as an Investigator in the Howard Hughes Medical Institute. He received a B.A. degree in chemistry from Lawrence University and a Ph.D. degree in molecular biology and biochemistry from Harvard. After postdoctoral research at the Medical Research Council Laboratory of Molecular Biology in Cambridge, England he joined the Yale faculty. He is a member of the U.S. National Academy of Sciences, the American Academy of Arts and Sciences and a Foreign Member of the Royal Society. He has received a number of awards, including the Rosenstiel Award for distinguished work in basic biomedical sciences, the Keio Medical Science Prize, the Gairdner International Award, the Connecticut Medal of Science and the 2009 Nobel Prize in Chemistry.
For the last three decades, research in the laboratory of Dr. Steitz has focused on obtaining insights into the molecular mechanisms by which the proteins and nucleic acids involved in the central dogma of molecular biology carry out gene expression from replication and recombination of the DNA genome to its transcription into mRNA followed by the various components associated with the translation of mRNA into protein. Not only are these processes fundamental to all life forms, but many of the macromolecules involved in these processes are known, or potential, targets for therapeutic drugs. In the 1980s, his lab established the structure of the catabolite gene activator protein and later its DNA complex, the structure of the first DNA polymerase and the first structure of an aminoacyl tRNA synthetase bound to tRNA. His lab is now continuing structural studies of all the components of the replisome. His studies of T7 RNA polymerase captured in many of its functionally important states - initiation, intermediate, elongation - as well as stages of nucleotide incorporation and provide the most complete picture of RNA transcription by an RNA polymerase. Perhaps the most significant insights have been derived from the atomic structure of the large ribosomal subunit. This structure proved that the ribosomal RNA is entirely responsible for catalyzing peptide bond formation and provided insights into how this mammoth RNA assembly is folded and functions as an enzyme. Most recently, research has focused on the structures of the 70S ribosome in complex with factors involved in various steps of the protein synthesis process. The ribosome is probably the major target of antibiotics. The many structures of the large subunit complexed with various different antibiotics determined at Yale have identified numerous different antibiotic binding sites near the site of protein synthesis. This information has been enormously facilitating to Rib-X Pharmaceuticals, Inc., now Melinta Therapeutics, in the development of new antibiotics that are effective against the antibiotic resistant bacteria.
Speakers
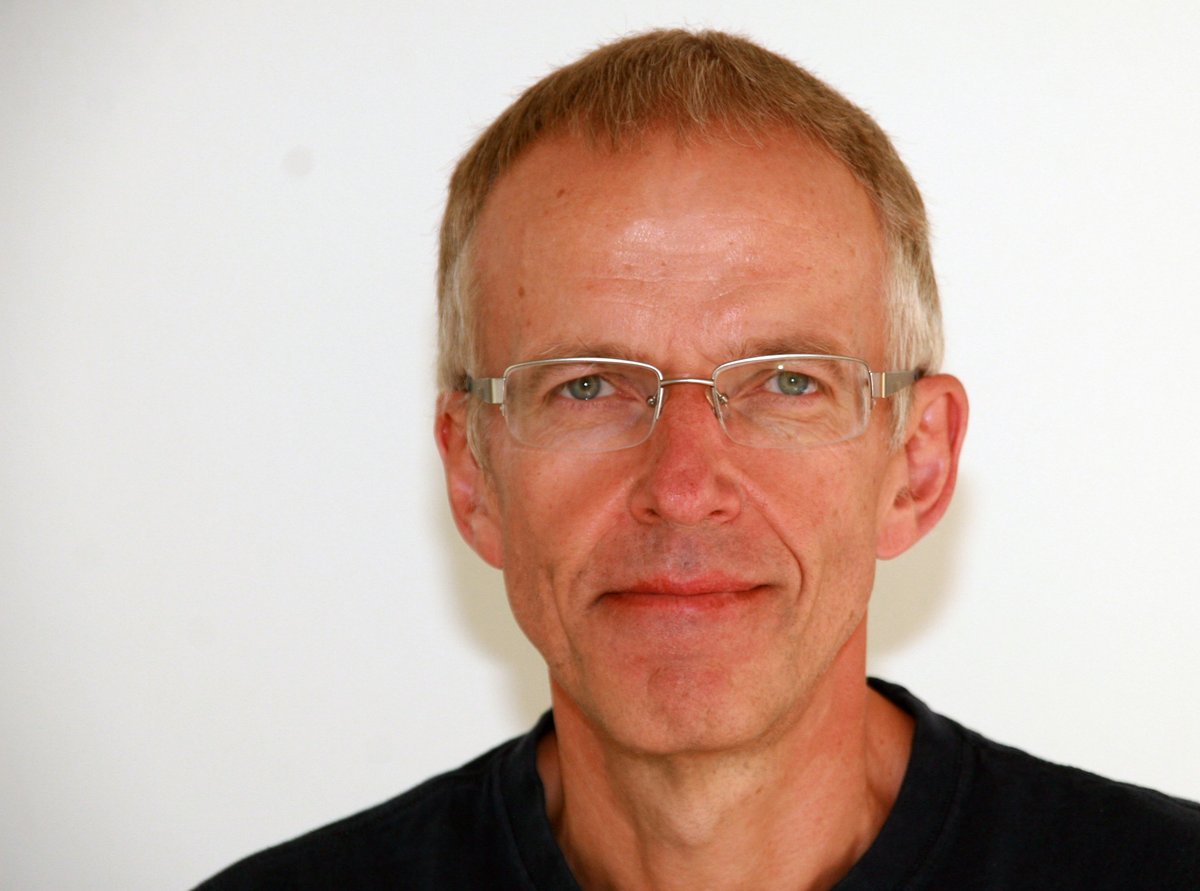
Jürgen Bajorath
Life & Medical Sciences Institute, Universität Bonn, DE
- Talk
Learning from Big Data at the Interface of Chemistry and Biology
The ‘big data’ concept plays an increasingly important role in many scientific fields. Big data involve much more than unprecedentedly large volumes of data, which is often not well understood. Different criteria characterizing big data must be carefully considered in computational data mining, as discussed in this presentation focusing on compound activity data. Compared to the situation in biology where big data are on the agenda for more than a decade, due to the availability of high-throughput genomics technologies, big data in medicinal chemistry are just beginning to emerge. For example, the ability of many drugs to specifically interact with multiple targets, termed promiscuity, forms the molecular basis of polypharmacology, a hot topic in drug discovery. Compound promiscuity analysis is an area that is much influenced by big data phenomena. Different results are obtained depending on chosen data selection and confidence criteria. As such, promiscuity analysis well illustrates the big data nature of compound activity measurements, which is challenging the practice of medicinal chemistry.
- About Jürgen Bajorath
Jürgen Bajorath received diploma and PhD degrees (1988) in biochemistry from the Free University in West-Berlin, Germany. He was a postdoc with Arnie Hagler at Biosym Technologies in San Diego. From 1990-2004, Jürgen held various positions in academia and the pharmaceutical industry in Seattle including a seven-year appointment at the Bristol-Myers Squibb Pharmaceutical Research Institute. In 1995, he became an Affiliate Professor at the University of Washington. In 2004, Jürgen was appointed Professor and Chair of the newly formed Department of Life Science Informatics at the University of Bonn. He continues to be an Affiliate Professor at the University of Washington. Jürgen is a member of numerous editorial and scientific advisory boards and an editor of the Journal of Medicinal Chemistry. His research focuses on chemoinformatics and the development of computational methods for chemical biology and drug discovery.
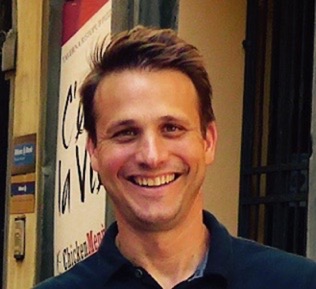
Matthew Bogyo
Stanford University, California, US
- Talk
New chemical probe technologies: applications to diagnostic imaging and drug discovery
Proteases are enzymes that often play pathogenic roles in many common human diseases such as cancer, asthma, arthritis, atherosclerosis and infection by pathogens. Therefore tools that can be used to dynamically monitor their activity can be used as diagnostic agents, as imaging contrast agents for intraoperative image guidance and for the identification of novel classes of protease-targeted drugs. In the first part of this presentation, I described our efforts to design and synthesize small molecule probes that produce a fluorescent signal upon binding to a protease target. We have identified probes that show tumor-specific retention, fast activation kinetics, and rapid systemic distribution making them useful for real-time fluorescence guided tumor resection and other diagnostic imaging applications. In the second half of the talk, I presented our recent advances using chemical probes to target a protease in the bacterial pathogen Clostridium difficile toxin proteins. Clostridium difficile infection (CDI) is a worldwide health threat that is typically triggered by the use of broad-spectrum antibiotics, which disrupt the natural gut microbiota and allow this Gram-positive anaerobic pathogen to thrive. The increased incidence and severity of the disease coupled with decreased response, high recurrence rates, and emergence of multiple antibiotic resistant strains have created an urgent need for new therapies. Through a targeted screen using one of our protease probes, we identified a number of potent inhibitors against a toxin derived cysteine protease. Importantly, one bioactive organo-selenium compound is currently in human clinical trials for a clinically unrelated indication. This drug showed activity in cell based assays and treatment in a mouse model of CDI that closely resembles the human infection confirmed a significant therapeutic benefit in the form of reduced disease pathology upon oral dosing of the drug. We are currently using selenium chemistry and molecular modeling to build more selective and potent inhibitors of the protease to be used as non-antibiotic therapeutics for the treatment of CDI. Taken together, this presentation highlighted multiple applications for chemical probes and presented examples in which our efforts have led to near-term clinical applications.
- About Matthew Bogyo
Dr. Bogyo is a Professor of Pathology and Microbiology and Immunology at Stanford University. He received his bachelor’s degree in Chemistry from Bates College in 1993 and a doctorate in Chemistry from Massachusetts Institute of Technology in 1997. He carried out a short post-doctoral fellowship at Harvard Medical School in 1998. Dr. Bogyo established an independent scientific career as a Faculty Fellow at the University of California, San Francisco, where he supervised a small laboratory of post-doctoral fellows and students. In 2001, Dr. Bogyo was hired to establish and direct the Chemical Proteomics Department at Celera Genomics focused on applying small molecule probes to the field of drug discovery. Dr. Bogyo then joined the Department of Pathology at Stanford University in July 2003 and was promoted to Associate Professor in 2009 and to full professor in 2013. His laboratory works on the development of new chemical probe technologies that are applied to the study the role of proteases in complex biological pathways associated with human disease. Dr. Bogyo has published over 200 primary research publications and currently serves on the Editorial Board of several prominent research journals. He was the President of the International Proteolysis Society from 2007-2009 and is the chair of the Gordon Research Conference on Proteolytic Enzymes and Their Inhibitors in 2018 and the Imaging in 2020 meeting in 2016. Dr. Bogyo is also a member of Stanford’s Comprehensive Cancer Center, the Molecular Imaging Program at Stanford (MIPS) and the ChEM-H Institute at Stanford. He is a consultant for several biotechnology and pharmaceutical companies in the Bay Area and is the co-founder of Akrotome Imaging, a small start up company developing imaging contrast agents for detection of surgical margins. He is the recipient of numerous awards including the Searle Scholar Award, the Terman Fellowship and the Burroughs Wellcome Fund Investigators in Pathogenesis award.
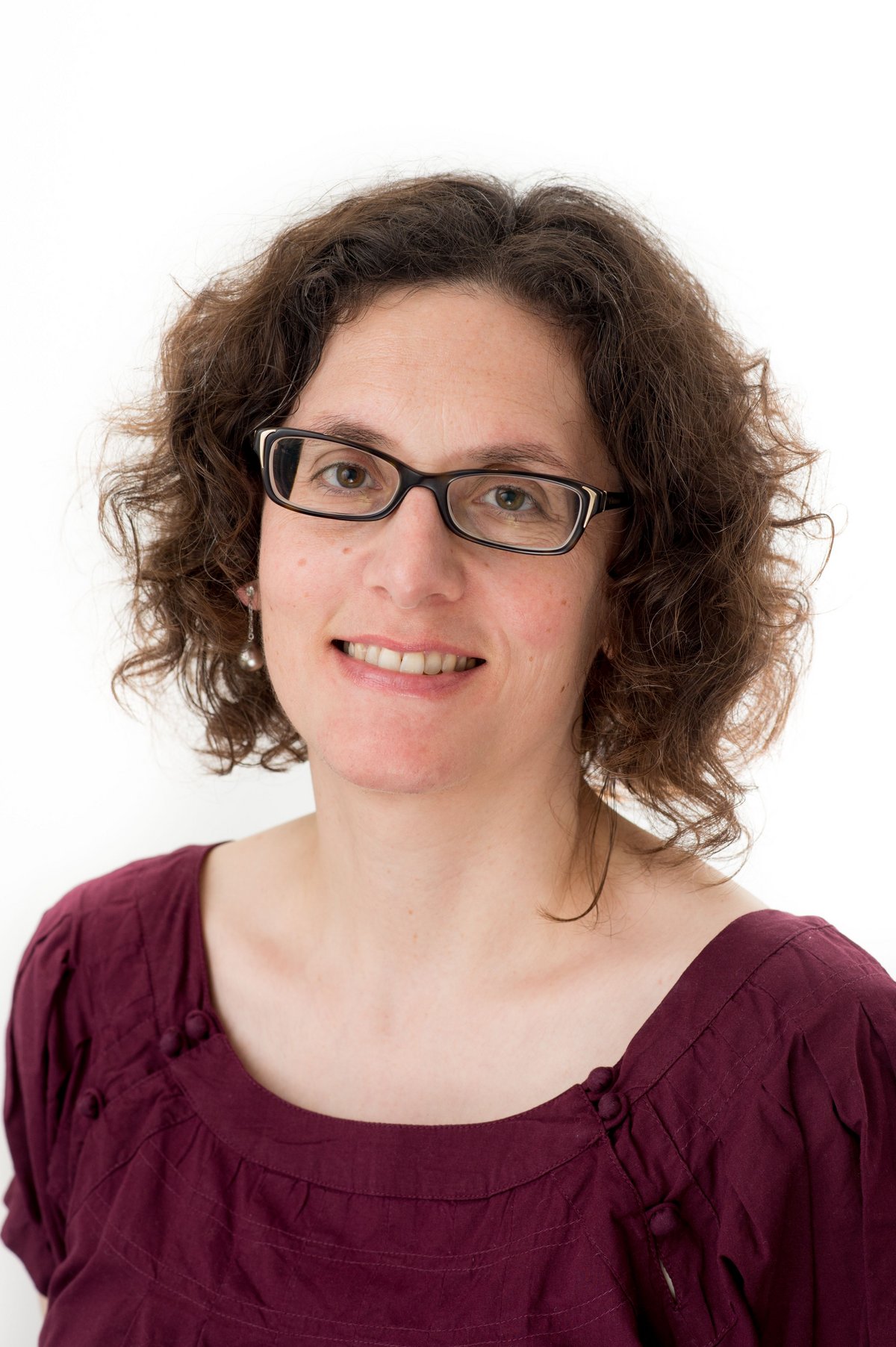
Ulrike Eggert
King’s College, London, UK
- Talk
A chemical approach to understanding cell division
How cells regulate and execute cytokinesis, the final step in cell division, remain major unsolved questions in basic biology. It has been challenging to study cytokinesis by traditional methods because it is a very rapid and dynamic process that occupies only a small portion of the cell cycle. New approaches are needed to overcome these barriers to deeper understanding, one of which is to develop probes that act rapidly and with high temporal control. We are in the process of creating a toolbox of small molecules that inhibit different proteins and pathways in cytokinesis.
I focused on our work to understand how membranes and membrane trafficking participate in cytokinesis. Although it is known that membranes are needed to seal daughter cells after severing, very little is known about whether (and how) specific lipids are involved in cytokinesis. Massive membrane rearrangements occur during cell division, suggesting that lipids play specific roles. We used mass spectrometry to determine if the lipidome changes in dividing cells and at a division site (the midbody) and found that only very specific lipids with specific side chains accumulate (Atilla-Gokcumen, Muro et al., Cell 2014). In parallel, we systematically used RNAi to knock down lipid biosynthetic enzymes and identified enzymes required for division, which highly correlated with lipids accumulated in dividing cells. Having determined the nature of lipids involved in cell division and their biosynthetic enzymes, the next steps are to understand their functions. To further investigate the lipids’ biological roles, we are using chemical biology and cell biology approaches.- About Ulrike Eggert
Ulrike Eggert is a chemical biologist who has been a Professor of Chemical Biology at King’s College London since 2015. She received her first degree in chemistry from the University of Oxford and her PhD in chemistry from Princeton University, working on vancomycin resistance with Professor Daniel Kahne. She then moved to Harvard Medical School as a Helen Hay Whitney Foundation postdoctoral fellow in the laboratory of Professor Tim Mitchison, where she first started research on cell division. In 2006, she started her independent career an Assistant Professor at the Dana-Farber Cancer Institute and Harvard Medical School and moved to King’s College London in 2011. Riki’s group uses chemical biology and cell biology approaches to study cell division at the process, pathway, protein and metabolite levels. A recent focus has been on understanding the roles lipids, and especially their side chains, play in processes driven by the cytoskeleton. Riki serves on several editorial and advisory boards and is an associate editor for Biochemistry. Her lab is currently funded by a Wellcome Investigator Award and an ERC Consolidator Grant.
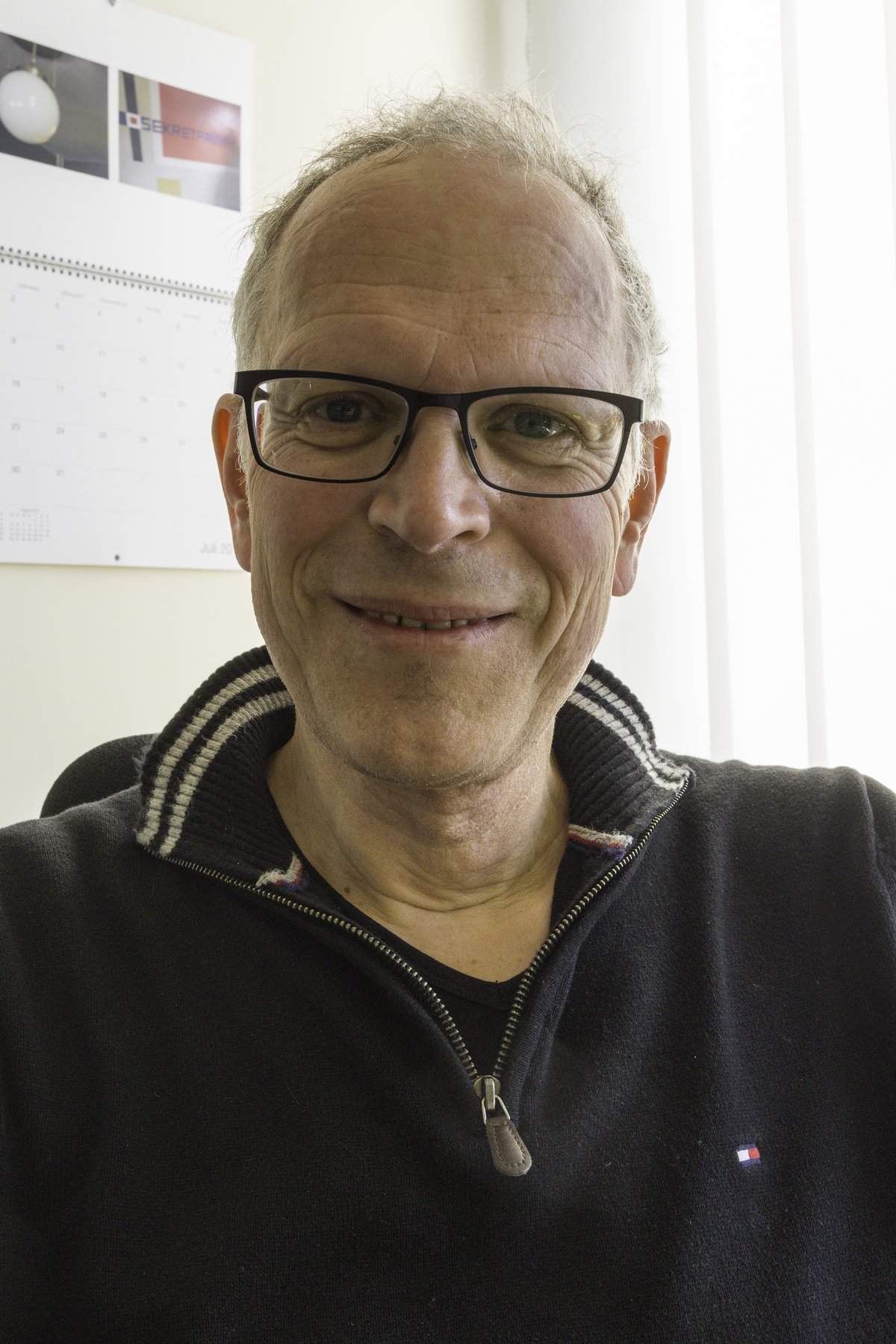
Michael Famulok
Life & Medical Sciences Institute, Universität Bonn, DE
- Talk
Chemical Biology of Aptamers and DNA Nanostructures
The in vitro selection of hugely complex libraries of single-stranded oligo(deoxy)nucleo-tides (ODNs) can lead to the enrichment of functional ODNs that recognize large varieties of target molecules. Library enrichments can be performed under many different conditions in vitro and in vivo, and on complex target molecules likes entire cells, tissues and organisms.
The first part of my presentation showed examples of in vitro selections performed on complex targets and examples illustrating the functional abilities of the resulting ODNs. The second part focused on DNA nanoarchitectures based on DNA origami structures, interlocked DNA molecules and bio-hybrid DNA architectures of designed and built-in functionality.
- About Michael Famulok
Michael Famulok studied Chemistry at the University of Marburg where he graduated in 1986 and obtained his Doctoral degree in 1989. From 1989-1990 he was postdoctoral fellow at the Department of Chemistry at MIT. From 1990-1992 he was postdoctoral fellow at the Department of Molecular Biology at Massachusetts General Hospital (Harvard Department of Genetics). He began his independent career at the LMU Munich in 1992. Since 1999 he is Professor for Biochemistry and Chemical Biology at the LIMES Institute at the University of Bonn. In 2002 he received the Gottfried-Wilhelm Leibniz Award of the DFG, and in 2010 an ERC advanced grant. Since 2013 he holds a Max-Plack-Fellowship at the Forschungszentrum Caesar in Bonn. Professor Famulok‘s research interests include aptamer technology, Chemical Biology, and functional DNA nanostructures.
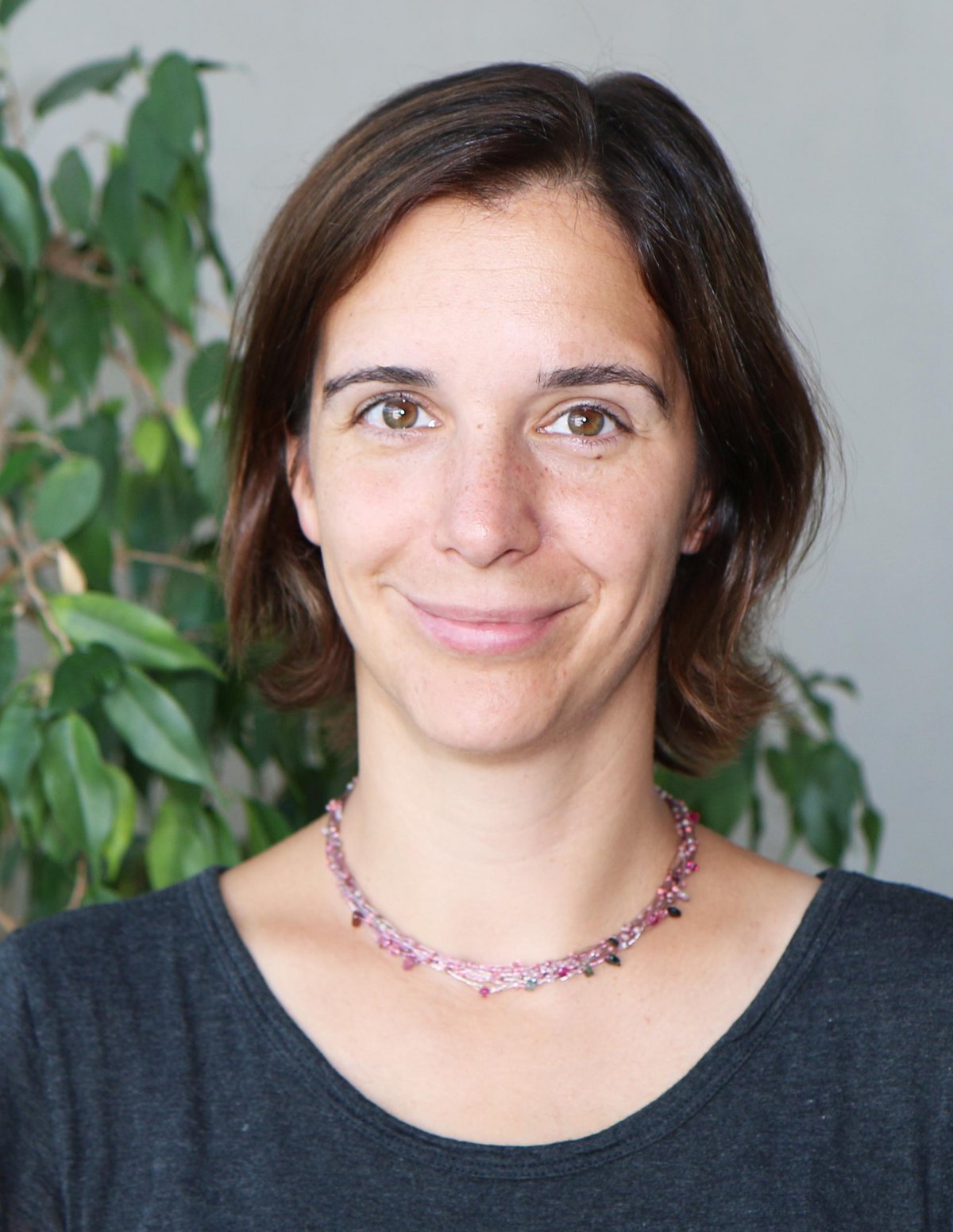
Dorothea Fiedler
Leibniz-Institut für Molekulare Pharmakologie, Berlin, DE
- Talk
Elucidating the functions and mechanisms of inositol pyrophosphate messengers with chemical tools
By integrating signaling cues with metabolic status, cells are able to modulate a range of processes according to their internal resources. The diphosphoinositol polyphosphates (PP-InsPs) are a unique group of highly phosphorylated messengers known to control glucose uptake, insulin signaling, and energy homeostasis, and thereby provide an important link between signaling and metabolic networks. It is thought that PP-InsPs exert their pleiotropic effects as allosteric small molecule regulators and via pyrophosphorylation of protein substrates, but most details in PP-InsP signaling have remained elusive due to a paucity of suitable reagents.
Our group is taking chemical approaches to uncover the molecular mechanisms in PP-InsP signaling. We have designed non-hydrolyzable PP-InsP analogues and have utilized these analogs for the affinity purification of inositol polyphosphate binding proteins. In parallel, we devised a method that provides easy access to pyrophosphopeptides. The pyrophosphopeptides were subsequently employed for the development of mass spectrometry-based detection of endogenous pyrophosphoproteins. The characterization of PP-InsP binding proteins and pyrophosphorylation substrates are now enabling us to annotate the complex PP-InsP signaling landscape.- About Dorothea Fiedler
After recieving a Diplom in Chemistry from the University of Wuerzburg, Dorothea Fiedler conducted her graduate work at the University of California, Berkeley, under the guidance of Kennth Raymond and Robert Bergman. Following postdoctoral work in Kevan Shokat’s lab at UCSF, she became an Assistant Professor in the Department of Chemistry at Princeton University in 2010. In 2015, she moved to the Leibniz-Institut for Molecular Pharmacology (FMP) and the Humboldt University, Berlin, where she continues to investigate cellular signaling pathways and metabolic networks, using a combination of chemical and biological approaches.
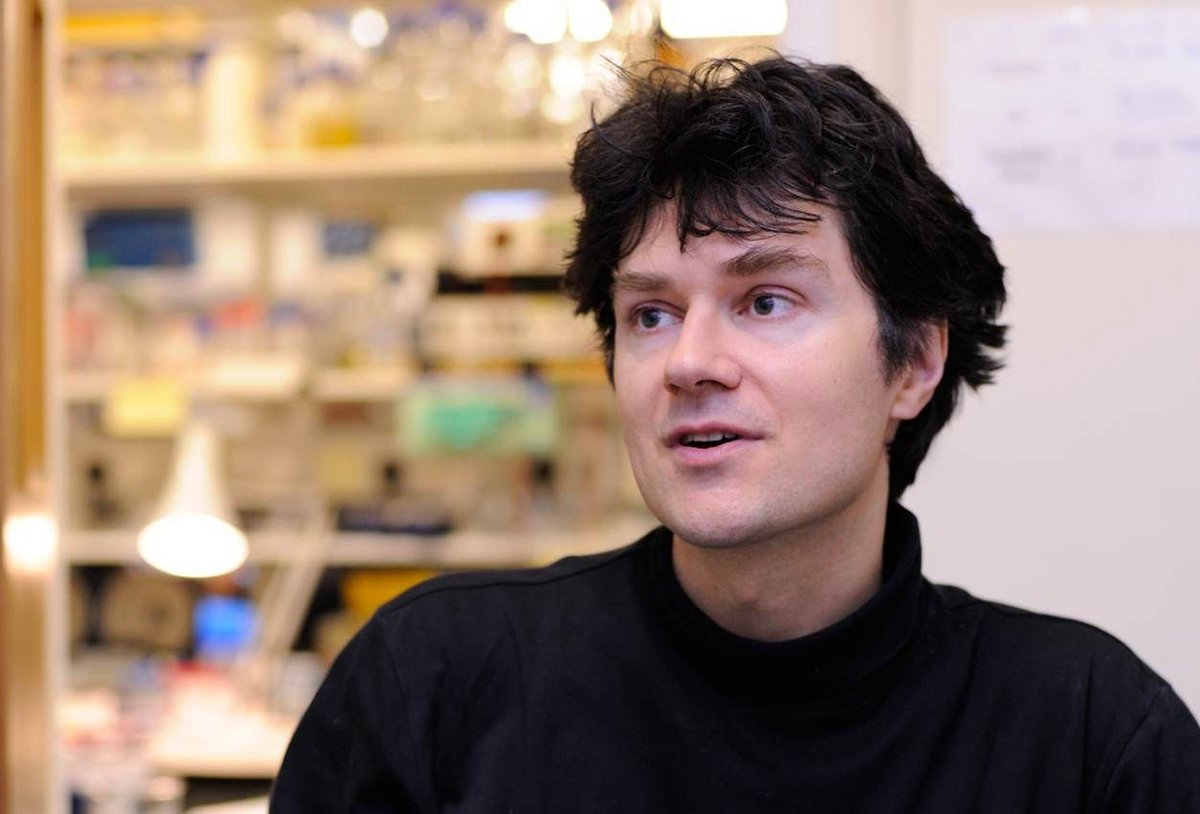
Philipp Holliger
Medical Research Council, Cambridge, UK
- Talk
Synthetic genetics: beyond DNA and RNA
Synthetic biology seeks to probe fundamental aspects of biological form and function by construction (i.e. resynthesis) rather than deconstruction (analysis). Synthesis thus complements reductionist and analytic studies of life, and allows novel approaches towards fundamental biological questions. We have been exploiting the synthesis paradigm to explore the chemical etiology of the genetic apparatus shared by all life on earth. Specifically, we ask why information storage and propagation in biological systems is based on just two types of nucleic acids, DNA and RNA. I presented recent progress on the development and application of strategies to enable the synthesis and replication of novel genetic polymers based on entirely synthetic backbones not found in nature, demonstrating information storage and propagation (i.e heredity) and Darwinian evolution of ligands (XNA aptamers) and catalysts (XNAzymes) [1,2]. Thus, heredity and evolution, two hallmarks of living systems, are not restricted to DNA and RNA but are likely to be emergent properties of al polymers capable of information storage.
- About Philipp Holliger
Philipp Holliger is a Program Leader at the MRC Laboratory of Molecular Biology in Cambridge. His research spans the fields of chemical biology and synthetic biology, has been published in major journals (Nature, Science, Cell etc.) and led to numerous patents. His recent work, describing a range of novel synthetic genetic polymers (XNAs) has featured in Scientific American’s 10 World Changing Ideas (2012). He is a member of EMBO and F1000 and has been a consultant to several successful biotech companies including Cambridge Antibody Technology / Medimmune, Solexa / Illumina, Domantis and GSK.
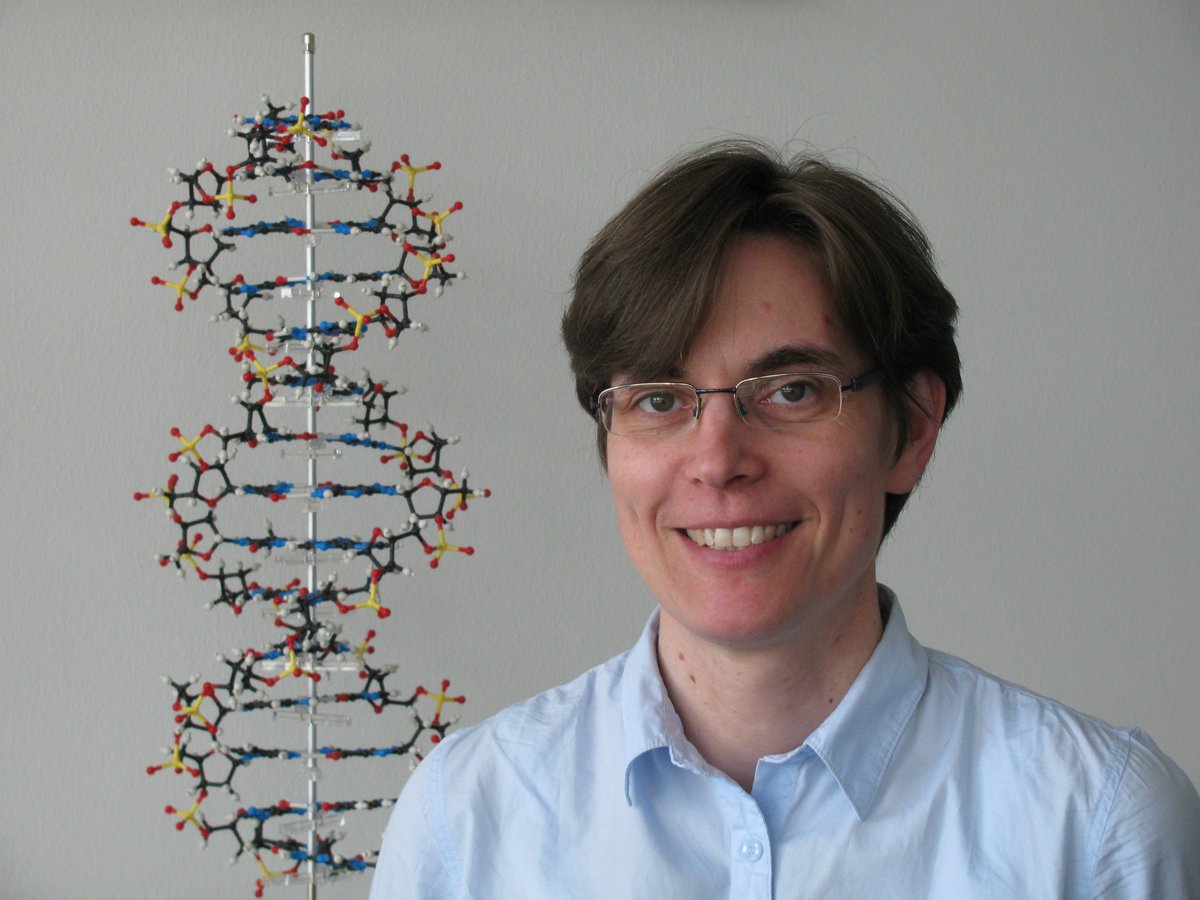
Claudia Höbartner
Georg-August-Universität Göttingen, DE
- Talk
Structure and Function of Catalytic DNA
Ribozymes catalyze chemical reactions that are vital for biological processes such as RNA splicing, tRNA biosynthesis and viral replication. Analogous catalytically active DNA molecules have not yet been found in nature. In the laboratory, new catalytic activities for DNA and RNA can be evolved by iterative selection and amplification of desired sequences from an initially random synthetic nucleic acid library. Numerous reactions can now be catalyzed by DNA, but details of the catalytic mechanisms are largely unknown, and until recently no structural information on any DNA enzyme was available.
Part of my research aims at a deeper understanding of nucleic acid catalysis and enhancing the catalytic abilities of DNA enzymes for practical applications. We focus on RNA-ligating DNA enzymes for the synthesis of site-specifically labeled RNA.[1] Combinatorial characterization methods revealed essential nucleotides in the catalytic core of the DNA,[2] and we found that certain DNA-catalyzed reactions are significantly accelerated by lanthanide ions.[1,3] The probing results enabled truncation of many DNA enzymes to essential sequence elements. A minimized DNA enzyme that catalyzes RNA ligation was successfully crystallized, which resulted in the first structure of a catalytic DNA.[4] The structure revealed fascinating insights into a complex active site made from DNA, and provided clues on the regioselectivity of bond formation and substrate preferences for the DNA-catalyzed reaction. Structure-guided mutagenesis allowed engineering of the catalyst for efficient ligation of previously inert RNA substrates.- About Claudia Höbartner
Claudia Höbartner studied chemistry in Vienna and Zürich, and earned a PhD degree from the Leopold Franzens University Innsbruck (Austria) in 2004. After postdoctoral research at the University of Illinois at Urbana-Champaign (UIUC), she returned to the University of Innsbruck, funded by the Hertha Firnberg career development program of the Austrian Science Fund (FWF). In 2008 she joined the Max Planck Institute for biophysical Chemistry in Göttingen as an independent research group leader. In 2014 she was appointed professor at the faculty of chemistry at Georg August University Göttingen. In summer 2017 she will establish her research group at the Institute for Organic Chemistry at the Julius Maximilians University Würzburg. Her research is focused on the biomolecular chemistry of natural and synthetic functional nucleic acids and was recognized by the European Young Chemist Award (EuCheMS, Silver medal, 2010), and research awards of the Peter and Traudl Engelhorn foundation (2011) and the Hellmut Bredereck foundation (2013). Since 2016 her research is also funded by an ERC consolidator grant.
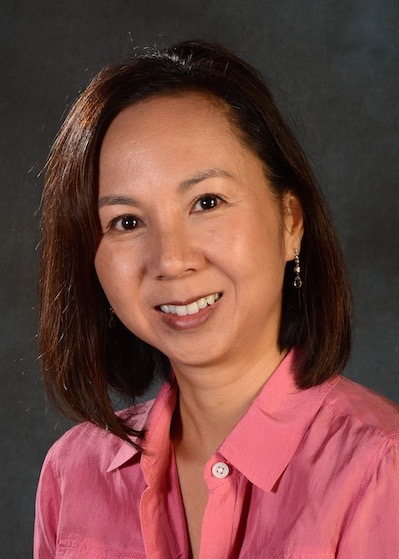
Linda C. Hsieh-Wilson
California Institute of Technology, Division of Chemistry and Chemical Engineering, US
- Talk
Harnessing Chemistry to Discover New Carbohydrate-Mediated Signaling Pathways in the Brain
Chemical neurobiology is rapidly evolving and providing insights into the molecules and interactions involved in neuronal development, sensory perception and memory storage. We described the synergistic application of organic chemistry and neurobiology to understand how specific carbohydrate molecules contribute to neuronal signaling in the brain. By combining synthetic organic and polymer chemistry, computational chemistry, proteomics studies, and in vivo biology, we have shown that these molecules actively participate in signaling events that underlie fundamental processes such as gene regulation, neuroregeneration, and the formation of neural circuits.
- About Linda C. Hsieh-Wilson
Dr. Linda Hsieh-Wilson is the Arthur and Marian Hanisch Memorial Professor of Chemistry at the California Institute of Technology. She received her B.S. degree magna cum laude in chemistry from Yale University in 1990. In 1996, she completed her Ph.D. in chemistry from the University of California at Berkeley, where she was a National Science Foundation predoctoral fellow in the laboratory of Professor Peter Schultz. In 1996, she moved to Rockefeller University to study neurobiology with Professor and Nobel Laureate Paul Greengard as a Damon Runyon-Walter Winchell postdoctoral fellow. Dr. Hsieh-Wilson joined the faculty at the California Institute of Technology in 2000, where she became an associate professor of chemistry in 2006 and full professor in 2010. She was appointed an investigator of the Howard Hughes Medical Institute in 2005, and in 2015, she was elected to the American Academy of Arts and Sciences. Her honors include the Beckman Young Investigator Award (2000), the Research Corporation Research Innovation Award (2000), an Alfred P. Sloan Fellowship (2003), the Eli Lilly Award in Biological Chemistry (2006), the Arthur C. Cope Scholar Award (2008), the Gill Young Investigator Award in Neuroscience (2009), and the Horace S. Isbell Award in Carbohydrate Chemistry (2014).
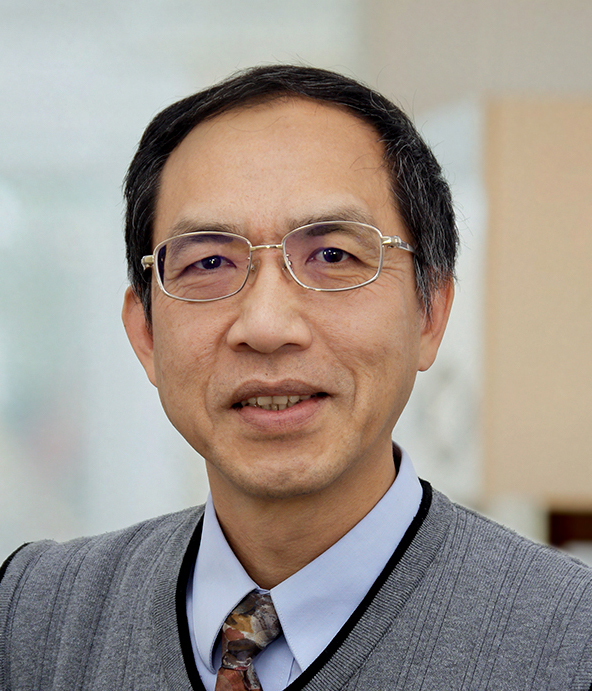
Shang-Chen Hung
Genomics Research Center, Academia Sinica, Taipei, Taiwan
- Talk
Probing Specific Interactions of Cell Surface Carbohydrates with Proteins
- About Shang-Chen Hung
Education and Positions held:
- Ph.D., Chemistry, National Tsing Hua University, 1992
- Postdoctoral Fellow, University of California at Berkeley, 1994-1995
- Postdoctoral Fellow, The Scripps Research Institute, 1995-1998
- Assistant Research Fellow, Institute of Chemistry, Academia Sinica, 1998-2002
- Associate Research Fellow, Institute of Chemistry, Academia Sinica, 2002-2005
- Associate Professor, Department of Chemistry, National Tsing Hua University, 2005-2006
- Professor, Department of Chemistry, National Tsing Hua University, 2006-2007
- Distinguished Professor, Department of Chemistry, National Tsing Hua University, 2007-2009
- Research Fellow, Genomics Research Center, Academia Sinica, 2009-2012
- Adjunct Professor, Department of Applied Chemistry, National Chiao Tung University, 2010-present
- Distinguished Research Fellow, Genomics Research Center, Academia Sinica, 2012-present
- Director, Genomics Research Center, Academia Sinica, 2016-present
- Acting Division Director of Biotechnology Incubation Center, Genomics Research Center, Academia Sinica, 2016-present
Honors:
- Postdoctoral Fellowship, Educational Ministry, 1994
- Young Scholar Research Publication Award, Academia Sinica, 2002
- Outstanding Young Investigator Award, The Chinese Chemical Society, 2002
- Outstanding Young Scholar Award, Tsing-Hua Fundation of Chemistry Technology, 2003
- Travel Grant Award for Young Chemists, IUPAC, 2003
- Mr. Tayou Wu Memorial Award, National Science Council, 2003
- Distinguished Research Award, National Science Council, 2004
- Outstanding Youth Medal, China Youth Corps, 2005
- Letcureship Award, International Conference on Cutting-Edge Organic Chemistry in Asia, 2006
- Yu-Ziang Hsu Scientific Paper Award, Far Eastern Y. Z. Hsu Science and Technology Memorial Foundation, 2008
- Distinguished Teaching Award, National Tsing Hua University, 2008
- Investigator Award, Academia Sinica, 2009
- Academic Publication Award, Chung-Shan Academic and Cultural Foundation, 2009
- Best Annual Article Award, The Chinese Chemical Society, 2009
- Distinguished Research Award, National Science Council, 2009-2011
- 17th Teco Award, Teco Technology Foundation, 2010
- NSC 2012 Outstanding Research Award, 2012
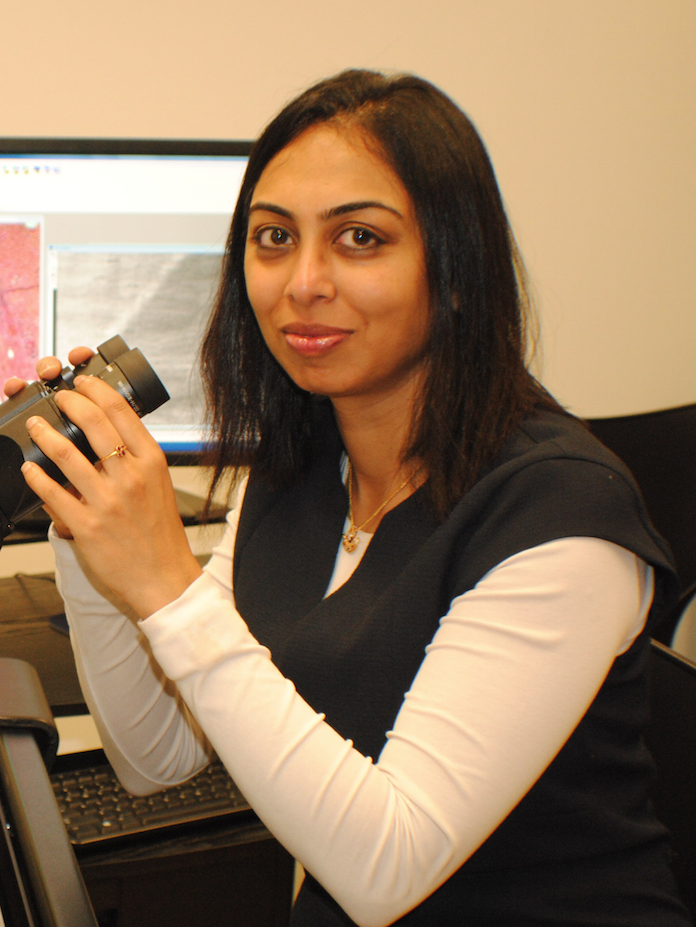
Yamuna Krishnan
University of Chicago, Illinois, US
- Talk
DNA nanodevices for cell specific cargo delivery
Due to its nanoscale dimensions and ability to self-assemble via specific base pairing, DNA is rapidly taking on a new aspect where it is finding use as a construction element for architecture on the nanoscale. Structural DNA nanotechnology has yielded architectures of exquisite complexity and functionality in vitro.1 However, till 2009, the functionality of such synthetic DNA-based devices in living organisms remained elusive.1 Work from my group the last few years has bridged this gap where, we have chosen architecturally simple, DNA-based molecular devices and shown their functionality in complex living environments.2,3 I described our recent work describing the design and application of a cell targetable icosahedral DNA nanocapsule4 to illustrate the potential of DNA based molecular devices as unique tools with which to interrogate living systems.
- About Yamuna Krishnan
Yamuna Krishnan, is a Professor and Brain Research Foundation Fellow of Chemistry and the Grossman Institute of Neuroscience at the University of Chicago.
Research in her laboratory spans supramolecular chemistry, nucleic acid biophysics, biochemistry, molecular biology, and cell biology. Her lab has pioneered the deployment of DNA nanodevices as quantitative fluorescent reporters of second messengers for in vivo imaging.
The youngest woman recipient of India’s highest scientific prize, the Shanti Swarup Bhatnagar Award, she was also featured by the journal Cell on their 40 under 40 list of young scientists who are shaping current and future trends in biology. She has also received the Wellcome Trust Senior Fellowship, the AVRA Young Scientist Award, Associateship of the Indian Academy of Sciences, the Innovative Young Biotechnologist Award, the INSA Young Scientist Medal, the Chemical Sciences Emerging Investigator by the RSC and the AWIS Chicago Innovator of the Year 2016.
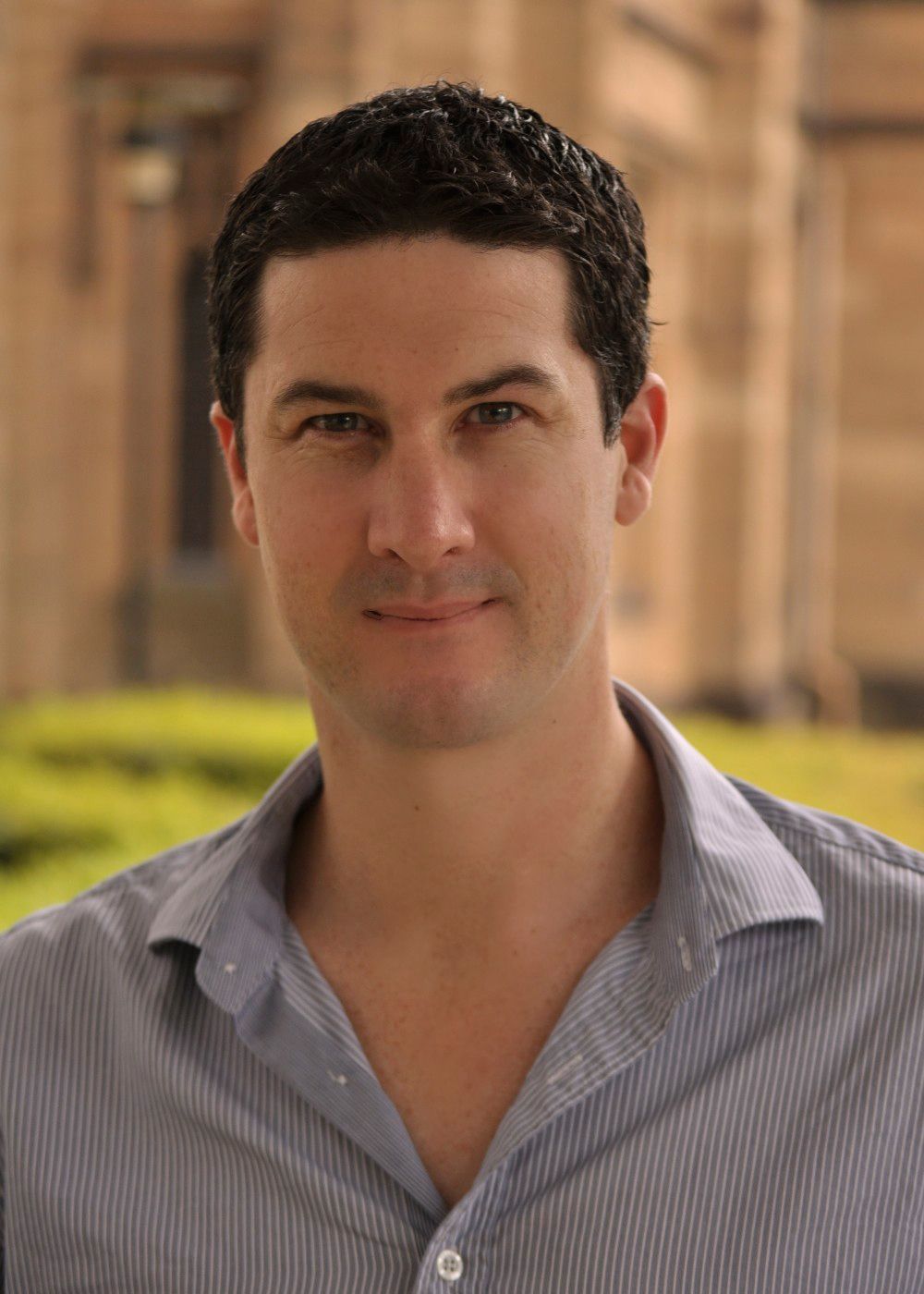
Richard J. Payne
The University of Sidney, AU
- Talk
New Methods for the Chemical Synthesis of Proteins Bearing Post-Translational Modifications
Post-translational modifications (PTMs) are ubiquitous chemical alterations that are made to proteins after ribosomal synthesis.1 The vast number of potential PTMs that can be installed on a specific protein leads to an explosion of structural diversity, with each isoform possessing potential variation in structure and function. This has led to significant interest in proteins bearing PTMs for use as pharmaceuticals.2 Unfortunately, the non-templated nature of the PTM process in vivo leads to heterogeneous mixtures of isoforms that hinders the ability to study the role of a single modification in a meaningful way. We have developed a number of new peptide ligation methods as tools for the efficient chemical assembly of peptides and proteins bearing homogeneous PTM patterns for the in depth interrogation of biological activity.3 Highlights of this work have been discussed in this seminar, including the development of peptide ligation and chemoselective desulfurization and deselenization chemistry at a range of proteinogenic amino acids4-7 The application of these technologies in the synthesis of several homogeneously modified bioactive proteins have also been highlighted as well as investigations of these proteins as therapeutic leads.
- About Richard J. Payne
Richard J. Payne graduated from the University of Canterbury, New Zealand, in 2002. In 2003, he was awarded a Gates Scholarship to undertake his PhD at the Department of Chemistry, University of Cambridge under the supervision of Professor Chris Abell. After his PhD, Richard moved to The Scripps Research Institute under the auspices of a Lindemann Postdoctoral Fellowship where he worked in the laboratory of Professor Chi-Huey Wong. In 2008, he moved to the University of Sydney as a Lecturer of Organic Chemistry and Chemical Biology within the School of Chemistry where he is currently Professor of Organic Chemistry and Chemical Biology and ARC Future Fellow. Prof. Payne’s research focuses on utilising the tools of synthetic chemistry to address problems of biological and medicinal significance. Current projects include the use of natural products for the development of new drug leads for tuberculosis and malaria. His lab has also developed a number of synthetic technologies for the ligation-based assembly of large polypeptides and proteins. These methodologies have been employed in the total chemical synthesis of a number of modified proteins to understand structure-function and for the elucidation of new drug leads for a range of diseases. He has been the recipient of several prizes as a result of his work including the Biota Medal for medicinal chemistry, the Rennie Memorial Medal (Royal Australian Chemical Institute), the Le Fevre Memorial Prize (Australian Academy of Science), the Athel Beckwith Lectureship, the Tregear Award in Peptide Chemistry, the Edgeworth David Medal (Royal Society of NSW), the MedChemComm Emerging Investigator Lectureship and the Prime Minister’s Prize for Physical Scientist of the Year (Malcolm McIntosh Prize).
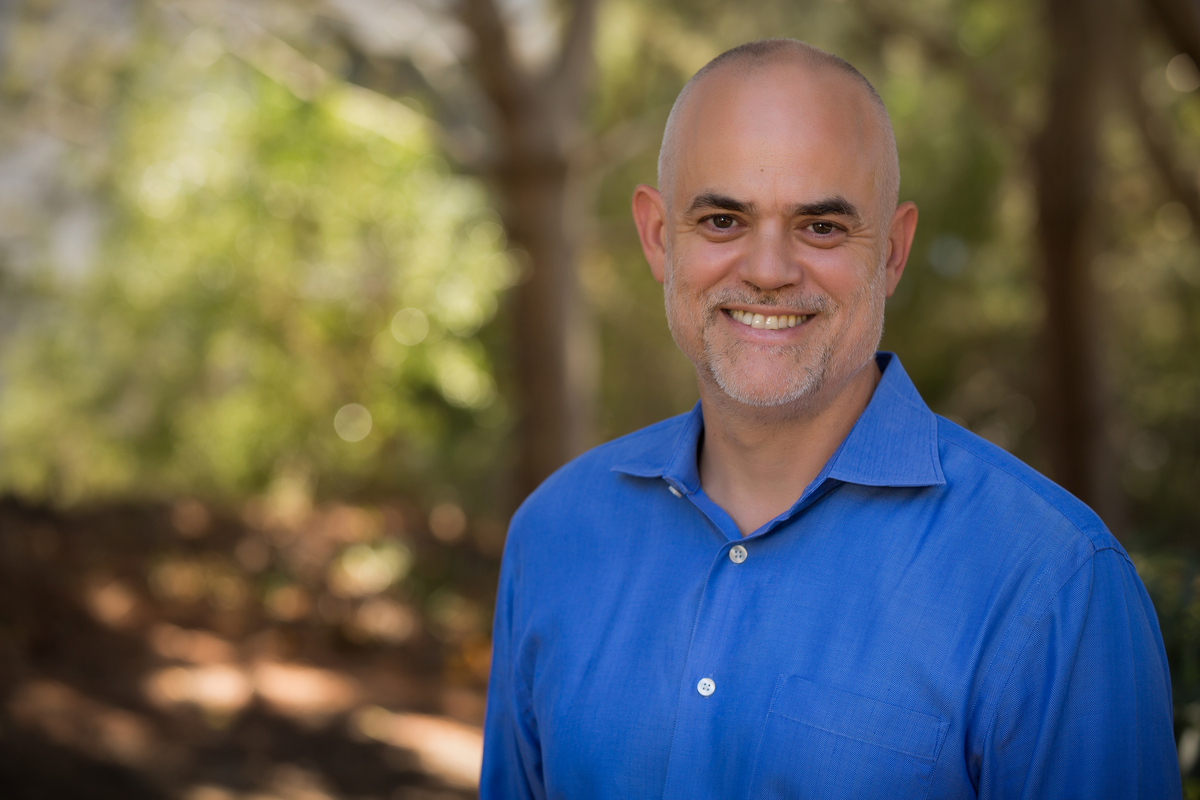
Floyd E. Romesberg
The Scripps Research Institute, San Diego, California, US
- Talk
A Semi-synthetic organism that stores and retrieves increased genetic infomation
Since the last common ancestor of all life on earth, the biological diversity has been encoded in a four letter, two base pair genetic alphabet. Expansion of the genetic alphabet to include a fifth and sixth letter than for a third, unnatural base pair not only has immediate utility for a number of applications, such as site-specific oligonucleotide labeling, but also serves as the foundation for an organism with an expanded genetic code. Toward this goal, we have examined a large number of different unnatural nucleotides bearing mainly hydrophobic nucleobase analogs that pair based on packing and hydrophobic interactions rather than H-bonding. Optimization based on extensive structure-activity relationship studies and two screens resulted in the identification of a class of unnatural base pairs that are well recognized by DNA and RNA polymerases. More recently, we have engineered E. coli to import the requisite unnatural triphosphates and shown that DNA containing the unnatural base pair is efficiently replicated, transcribed, and translated within the cell, resulting in the first semi-synthetic organism that stores and retreives increased information.
- About Floyd E. Romesberg
Dr. Romesberg has managed a research group at The Scripps Research Institute since 1998. His group uses a broad range of techniques, including non-linear optical spectroscopy, organic chemistry, microbiology, and genetics, to study different aspects of evolution. This work has been described in more than 100 peer-reviewed publications and has been funded by the National Institutes of Health, National Science Foundation, Office of Naval Research, and the Defense Advanced Research Projects Agency. Dr. Romesberg is best known for developing unnatural base pairs for the expansion of the genetic alphabet and is also working to develop novel antibiotics, as well as to understand how evolution tailors protein dynamics and how cellular stress and DNA damage induce cell-cycle checkpoint responses and mutations. Dr. Romesberg is also the scientific founder and a member of the board of directors of Synthorx. Prior to Synthorx, he served as the scientific co-founder and a member of the board of directors of RQx Pharmaceuticals (acquired by Genentech) and Achaogen. Dr. Romesberg received a B.S. in chemistry from The Ohio State University and a Ph.D. in physical organic chemistry from Cornell University.
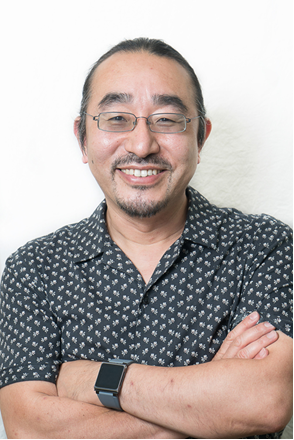
Hiroaki Suga
University of Tokyo, JP
- Talk
A RaPID way to discover pseudo-natural peptides for therapeutic uses
The genetic code is the law of translation, where genetic information encoded in RNA is translated to amino acid sequence. The code consists of tri-nucleotides, so-called codons, assigning to particular amino acids. In cells or in ordinary cell-free translation systems originating from prokaryotes, the usage of amino acids is generally restricted to 20 proteinogenic (standard) kinds, and thus the expressed peptides are composed of only such building blocks. To overcome this limitation, we recently devised a new means to reprogram the genetic code, which allows us to express non-standard peptides containing multiple non-proteinogenic amino acids in vitro. This lecture described the development in the genetic code reprogramming technology that enables us to express natural product-inspired non-standard peptides and pseudo-natural products. The technology involves (1) efficient macrocyclization of peptides, (2) incorporation of non-standard amino acids, such as N-methyl amino acids, and (3) reliable synthesis of libraries with the complexity of more than a trillion members. When the technology is coupled with an in vitro display system, referred to as RaPID (Random non-standard Peptide Integrated Discovery) system as a novel “molecular technology”, the libraries of natural product-inspired macrocycles with a variety ring sizes and building blocks can be screened (selected) against various drug targets inexpensively, less laboriously, and very rapidly. This lecture discussed the most recent development of their technology and therapeutic applications toward drug discovery innovation.
- About Hiroaki Suga
Hiroaki Suga is a Professor of the Department of Chemistry, Graduate School of Science in the University of Tokyo. He was born in Okayama City, Japan in 1963. He received his Bachelor of Engineering (1986) and Master of Engineering (1989) from Okayama University, and Ph. D. in Chemistry (1994) from the Massachusetts Institute of Technology. After three years of post-doctoral work in Massachusetts General Hospital, he was appointed as a tenure-track Assistant Professor in the Department of Chemistry in the State University of New York at Buffalo (1997) and promoted to the tenured Associate Professor (2002). In 2003, he moved to the Research Center for Advanced Science and Technology in the University of Tokyo as an Associate Professor, and soon after he was promoted to Full Professor. In 2010, he changed his affiliation to the Department of Chemistry, Graduate School of Science. His research interests are in the field of bioorganic chemistry, chemical biology and biotechnology related to RNA, translation, and peptides. He is also a founder of PeptiDream Inc. Tokyo, a publicly traded company at the first section of the Tokyo Stock Exchange, which has many partnerships with pharmaceutical companies in worldwide.
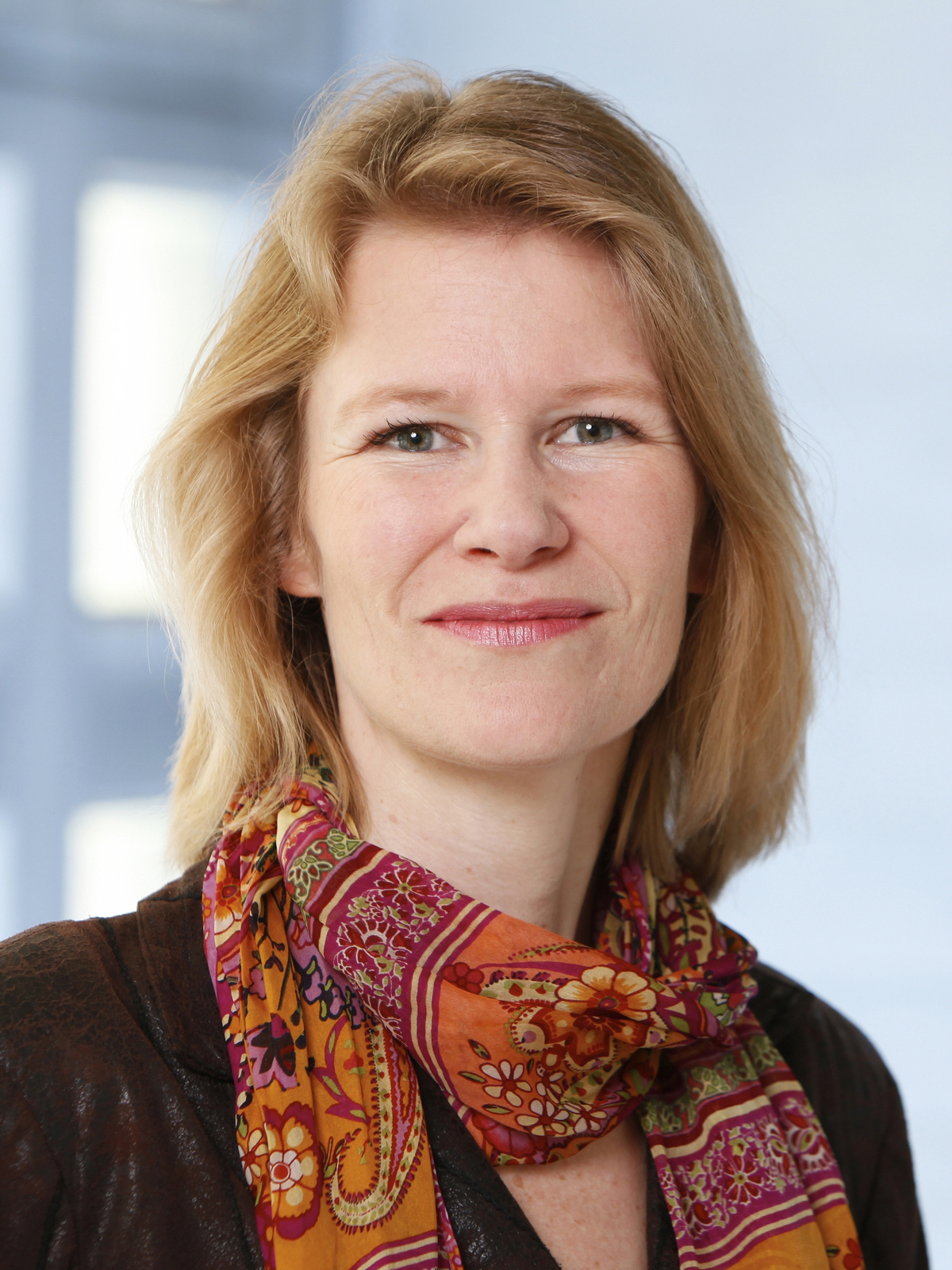
Helma Wennemers
ETH Zürich, CH
- Talk
Controlling Supramolecular Assemblies with Proline-Rich Scaffolds
Self-assembly and selective recognition events involving proteins are critical in nature for the function of numerous different processes, for example, catalysis, signal transduction or the controlled formation of structural components such as bones. My group is intrigued by the question whether also peptides with significantly lower molecular weights compared to proteins can fulfill functions for which nature evolved large macromolecules. Specifically we ask whether peptides can serve as effective asymmetric catalysts, templates for the controlled formation of metal nanoparticles, hierarchical supramolecular assemblies, synthetic collagen based materials, or tumor targeting vectors.
The lecture illustrated the value of distance-controlled molecular templates for the development of supramolecular assemblies.- About Helma Wennemers
Helma Wennemers studied chemistry at the Johann-Wolfgang-Goethe University in Frankfurt before moving to Columbia University, New York where she received her PhD degree for studies with W. Clark Still in 1996. Following postdoctoral studies at Nagoya University with Hisashi Yamamoto (1997–1998), she joined the faculty of Basel University as the Bachem-endowed Assistant Professor in 1999. She was promoted to Associate Professor (2004) at the University of Basel before moving to ETH Zurich in the fall of 2011 where she is Professor of Organic Chemistry.
Her research focuses on the development of small molecules with functions that are fulfilled in nature by large macromolecules. She utilizes the power of organic synthesis to access functionalities that nature might have not had in the repertoire of building blocks. The focus is both on practical applications and an understanding of the properties on the molecular level. This scope includes the development of bioinspired asymmetric catalysts, functionalizable collagen, the controlled formation of metal nanoparticles as well as the use of molecular scaffolds for applications in supramolecular and biological chemistry (e.g., cell-penetrating peptides, and tumor targeting).
Helma received several recognitions for her research, including Pedler Award (2016), JSPS Distinguished Lectureship (2016), Leonidas Zervas Award (2010), David Ginsburg Lectureship (2010), Holger Erdtman Lectureship (2010) and most recently Chemical Record Lectureship (2017), Calvin Lecture, University of California Berkeley (2017), and the Inhoffen Medal (2017).
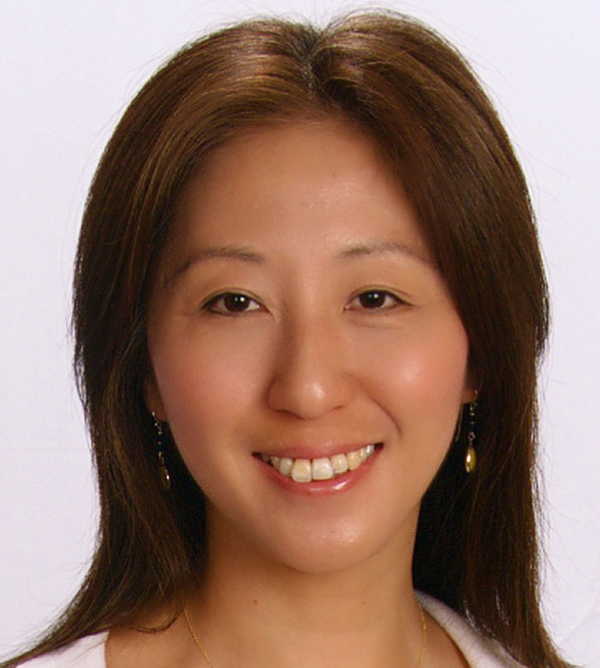
Wei Yang
National Institutes of Health (NIH), Bethesda, Maryland, US
- Talk
A new paradigm for catalysis of nucleotidyl-transfer reactions
Enzyme catalysis has been studied for over a century. How it actually occurs has
not been visualized until recently. By combining in crystallo catalysis with X-ray
diffraction analysis of reaction intermediates, we have observed the processes of
DNA synthesis and RNA at unprecendented atomic detail. Contrary to the
canonical view that enzyme-substrate complexes and transition states have
identical atomic composition and catalysis occurs by a two-metal-ion mechanism,
we have discovered that it is essential for a third divalent cation to be captured en
route to product formation. Unlike the canonical two metal ions, which are
coordinated by DNA polymerases and nucleases, this third metal ion is free of
enzyme coordination and is liganded by nucleic acid substrate/product only. Its
location between the α- and β-phosphates of dNTP in the case of DNA synthesis
suggests that the third metal ion may drive phosphoryltransfer from the leaving
group opposite to the 3´-OH nucleophile. Experimental data indicate that binding
of the third metal ion may be the rate-limiting step in DNA synthesis, and the free
energy associated with metal-ion binding can overcome the activation barrier to
the DNA synthesis reaction.- About Wei Yang
Wei Yang is a structural biologist specializing in studies of DNA replication, repair and recombination. She has been a senior scientist in the Laboratory of Molecular Biology of the National Institute of Diabetes and Digestive and Kidney Diseases, National Institutes of Health, since 1995. She was born in Shanghai, China and is a naturalized US citizen. She began her undergraduate studies of biochemistry at Fudan University in Shanghai before transferring to SUNY at Stony Brook to complete her B.A. degree. She received her Ph.D. in Biochemistry and Molecular Biophysics from Columbia University and held postdoctoral fellowships both at Columbia and Yale Universities. She received the Dorothy Crowfoot Hodgkin Award from the Protein Society in 2011 and Mildred Cohn Award in Biological Chemistry from ASBMB in 2017. She has been an elected member of the National Academy of Sciences (NAS) since 2013 and the American Academy of Arts and Sciences (AAAS) since 2015.
Dr. Yang’s current research centers on understanding the molecular mechanisms of DNA replication, repair and recombination with a focus on translesion DNA synthesis, genetic rearrangement and mismatch and nucleotide excision repair of damaged DNA. Her studies employ a multifaceted approach for analyzing the structures and activities of protein and nucleic acid molecules, including X-ray crystallography, electron microscopy, molecular biology, enzymology, and protein and nucleic acid chemistry. She has shown how different repair processes and directional motions are coupled to the energy supply of the cell, and how lesions in DNA can be avoided and bypassed by specialized synthetic enzymes. Her research group has described how the substrate specificity of many enzymes is determined by a stringent requirement for a pair of metal ions held in a particular conformation. Recently her team made use of crystallographic techniques to obtain the first atomic-resolution and time-resolved picture of DNA synthesis, discovering transiently associated metal ions that are essential for the addition of each new nucleotide unit.